Portions of the text below have been excerpted from the following NALMS publications:
Carlson, R.E. and J. Simpson. 1996. A Coordinator’s Guide to Volunteer Lake Monitoring Methods. North American Lake Management Society. 96 pp.
Section 314 of the Clean Water Act requires that all lakes of the Nation be classified according to their “eutrophic” character. Federal requirements for Section 305(b) of the same Act requires that “fishable” and “swimmable” goals be constructed for each state. Federal requirements such as these have resulted in the construction and use of numerous classification schemes (Brezonik and Shannon 1971; Carlson 1977; USEPA. 1974; Vollenweider and Kerekes 1980). These indices vary considerably in approach to classification and in the variables used for classification. All of these indices are “trophic state indices.” They are predicated on the ideas that: (1) the “trophic state” of a lake is an important water quality variable; and (2) indices can determine not only the trophic character of the water body, but also if it is usable for fishing or swimming.
If trophic state classification is as valuable as the interest in it suggests, then it would be advantageous if volunteers could aid in the collection of classification data. Often, however, people perceive the trophic state concept and the ensuing classification systems as too complex for volunteers. This is simply not true. There are simple approaches to trophic state classification that are not only easy to use, but actually closer to the original intent of the concept. The purpose of this chapter is to give some perspective and guidance to the coordinator with regard to trophic classification systems and to suggest how they can be used in volunteer programs.
Trophic State, The Concept
To understand the complexity and confusion associated with the present concept of trophic state, it is necessary to begin with a brief excursion into the history of “trophic state.” We’ll tease apart some of the numerous strands that are woven together into the present concept, and suggest that some of the strands are not as important to the concept as others, and therefore, can and should be discarded.
Einar Naumann, a Swedish limnologist at the University of Lund, Sweden, first developed what we now think of as the trophic state concept in the early part of this century (Naumann 1919). Although trophic state is usually associated with his classification system, it is important to realize that Naumann saw the classification as an artificial outgrowth of a biological reality.
From a 1929 paper, Naumann’s concept of trophic state can be summarized with four clear propositions about the relationship of the watershed to the functioning of a lake:
- The amount of algae (production) in a lake is determined by several factors, primarily by the concentration of nitrogen and phosphorus.
- Regional variations in algal production correlate with the geological structure of the watershed; lakes in agricultural, calcareous regions were greener than lakes in forested, granitic watersheds.
- The amount of production in a lake affects the lake biology as a whole.
- There are certain evolutionary (ontological) connections between lakes of the various types; lakes become more productive as they age.
The trophic state concept, as described by Naumann, began with the chemistry of the watershed. Nutrients and other chemicals from the watershed, together with factors such as temperature and light, affected the abundance of algae (production) in the lake. Production, in turn, affected the entire biological structure of the lake. A moment’s reflection makes us realize that this is a very contemporary interpretation of the matrix of factors affecting a lake’s biology.
These statements are neither some fuzzy speculation nor rigid dogmatism about how lakes should be classified; instead, they are specific, testable statements about connection between watershed characteristics and the biology and the ontogeny of lakes. In Naumann’s statements are the beginnings of ideas of nutrient loading, biomass-phosphorus relationships, and potential changes in trophic status as a lake ages.
What is also remarkable about Naumann’s ideas at this time is his insistence that there should be a regional approach to the study of limnology. He wrote in 1929:
“The advancement of the science of water-types – and of regional limnology as a whole – is of course dependent upon the collection and comparison of as abundant data as possible from different countries…In this respect our special journals could greatly further the advance of limnology by making it an absolute condition for publication that contributions should provide the data in question without which, indeed, most such communications are quite worthless for comparative purposes.”
In the sixty years after Naumann, limnologists retreated from comparing lakes on a regional basis. It might be well for coordinators of volunteer programs to consider that they are the hope for regional limnology that Naumann envisioned so many years ago.
Trophic State, The Classification System
Einar Naumann also developed what we now think of as trophic state terminology, using terms that Weber (1907, as cited in Hutchinson 1969) used to classify the nutrient content of bogs. According to Weber, oligotrophic bogs were raised bogs where the nutrients had leached out, while eutrophic bogs were sunken, and nutrients accumulated in them. Thus the idea that oligotrophic means “poorly-fed” and eutrophic means “well-fed” originated from the nutrient condition of bogs, not lakes (Hutchinson 1969).
Naumann used the terms, but not necessarily Weber’s concept, in classifying lakes. He based his original trophic classification on the “quantitative production of phytoplankton” (Naumann 1929). Oligotrophic lakes were those with low production, “never leading to a coloring or even a clouding of the water.” In eutrophic lakes, production attains very high values, “the water being, for the most part, very strongly clouded or even completely colored.”
Naumann related these trophic lake types to the physical and chemical factors that affect production. These factors included temperature (with which he divided the world into Arctic and Alpine, Temperate, and Tropical zones), light, and chemical factors (calcium, humic content, nitrogen and phosphorus, iron, pH, oxygen, and carbon dioxide). He divided the possible range of values for each of these factors, which he called milieu-spectra, into low (oligotypus), medium (mesotypus) and high (polytpus) “size-classes” or groups. For example, an oligotrophic lake might have oligotypus values of N and P and oligo or mesotypus levels of humus (Naumann 1929). Each of Naumann’s original lake types may have been based on a measure of production, but he combined the measure of production with a description of the factor (or factors) that were related to that production. However, he emphasized that trophic classification was based on production, not the factor determining that production. He considered nitrogen and phosphorus to be the primary determinants of production.
Table 7.1 Trophic lake types suggested by Naumann | |
Lake Type | Characteristics |
Oligotrophy | Lakes with low production associated with low nitrogen and phosphorus. |
Eutrophy | Lakes type with high production, associated with high nitrogen and phosphorus. |
Acidotrophy | Lake type with low production, with pH values less than 5.5. |
Alkalitrophy | Lake type with low production, associated with high calcium concentrations. |
Argillotrophy | Lake type with low production, associated with high clay turbidity. |
Siderotrophy | Lake type with low production, associated with high iron content. |
Dystrophy | Lake type with low production, associated with high humic color. |
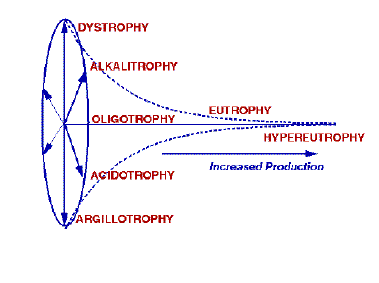
Figure 7.1. A representation of the basic typology of Naumann’s wassertypologie. We have modified it somewhat by implying that the lakes radiating from oligotrophy cannot be found in eutrophic or hypereutrophic nutrient or productivity conditions. Naumann did not imply this in his original classification.
As more lakes were studied, it became evident that, although some of these lakes had production as low as in oligotrophic lakes, the biological community was distinctly different from the typical oligotrophic lake. Often these lakes were at the extremes of non-nutrient, chemical axes. Naumann considered these to be new types when production was largely affected by factors other than nutrients. The dystrophic lake type, actually described by Thienemann (1921), had low N and P, but moderate to high content of humus material. Argillotrophic lakes had low productivity but the primary trophic factor was the abundance of clay in the water. Acidotrophic lakes, found at pH values less than 5.5, had as low productivity as oligotrophic lakes, but a different biological community (Naumann 1931; 1932). A list of many of Naumann’s lake types are given in Table 7.1. A representation of how we envision Naumann’s concept of the relationship between production and the trophic types is illustrated in Fig. 7.1.
In Germany, August Thienemann simultaneously developed a classification scheme based on the species of benthic organisms in lakes and the importance of the hypolimnetic oxygen concentration on their species composition (Thienemann 1921). It must have seemed reasonable at the time that these two classifications could be joined, because Naumann’s eutrophic lakes also lacked oxygen in the hypolimnion and had distinct benthic fauna (Thienemann 1921). For a while, the marriage of these systems seemed perfect and the study of trophic classification grew rapidly (Rodhe 1975).
As often happens with classifications of Nature, more and more lakes were found with characteristics of more than one of the established types. Hypolimnetic oxygen was supposed to be a primary discriminator between oligotrophy and eutrophy, but it was found that hypolimnetic oxygen was not solely dependent on the biological production of the lake, but was also affected by temperature and the morphometry of the lake basin. Tropical lakes had low algal biomass and productivity but still had anoxic hypolimnia. Lakes with small hypolimnia exhibited anoxia despite low productivity.
Encounters with situations such as these intensified the splitting of the terminology, generating types such as morphometric oligotrophy (Lundbeck 1934, as cited in Hutchinson 1973) for deep lakes with mesotrophic to eutrophic production but, because of the large hypolimnetic volume, still had oxygen in the hypolimnion. Järnefelt (1932) proposed the term “mixotrophy” to designate lakes that had characteristics of both oligotrophy and eutrophy. Thienemann (1926) went so far as to state that the lake typology was only applicable in the temperate regions. The trophic state terminology became increasingly cumbersome as the number of recognized lake types increased. Classification became difficult, and most of the terminology finally lapsed into disuse.
Trophic State Classification in Retrospect
The seeds for the failure of the Naumann-Thienemann trophic classification were sown almost at its inception. The problems with the classification were not that a system was not needed nor that the variables chosen to classify lakes were incorrect. Instead, problems occurred because: (1) the classifications tried to incorporate all of the variables, both causal and the biotic and abiotic consequences, into a single classification; and (2) people assumed that there existed distinct sets of lakes that could be easily classified.
Naumann’s original idea to classify lakes on the basis of production (biomass) had both practical and theoretical validity. Production, however he defined it, was a single axis that he divided into convenient groupings (high, medium, low production) and all aquatic bodies could be classified into these groups. Naumann could map the regional variation in production and could then ask valid scientific questions as to what factor or factors might be causing observed differences in production.
Problems began when Naumann linked the production classification with the causal factors of that production. Again, a useful classification scheme could be constructed based solely on the factors that affected or limited production. Indeed, present day trophic classifications based on phosphorus are remnants of Naumann’s production factor classification. The classification could have been univariate, based on the single factor that limited production (nutrients: Oligotrophy – Eutrophy, clay: Argillotrophy, humic color: Dystrophy, etc.). Instead, Naumann chose to incorporate all possible factors affecting production into the classification. He dealt with combinations of factors by combining the names. For example, an acid lake with high humic coloring and iron would be called Dys-Acido-Sidero-trophic (Naumann 1932). If he had found a lake that was high in algae as well, he might have added eutrophic to that name.
If the terminology was not cumbersome enough, production, the supposed primary standard of trophic classification, was instead the primary cause of the proliferation problem. If Naumann wanted to classify a lake on the basis of production and the factor associated with that production, then a new classification term had to be erected with each new potential factor affecting production. If he had known of biological growth limitations by grazing, salinity, water residence time, or morphometric factors, the classification scheme may have grown even further.
When biological structure, such as the benthic invertebrate community, was added to the classification scheme, the problems grew even more. Although the biological community is an important aspect of limnological ecology, it added a third level of complexity to the classification. Consequently, the scheme had to consider factors of limitation, production, and now, biological structure. However, production is only one of several factors affecting the biological community composition. Forcing biological structure into the production index denied the importance of those other factors. The result was a classification scheme that could not predict structure from production alone, yet structure was used to predict production.
An additional problem came when hypolimnetic oxygen concentration was added to the classification. As with biological structure, hypolimnetic oxygen is also affected by factors other than production. When given equal status, the resulting classification could be affected by temperature or basin morphometry as much as by biological production.
The net result was (and is) a classification system that is “trophic” in name only. It involved the degree of production, abiotic elements that can affect that production, biological structure, and hypolimnetic oxygen concentration. Thus even in its most “developed” form, the classification could only be a listing of general characteristics of generalized lakes. As a result the classification degenerated into a series of pigeon holes into which lakes were forced rather than fit.
Today trophic state terms such as oligotrophy and eutrophy are still commonly used, and terms such as dystrophy and argillotrophy can occasionally be found in the literature. Unfortunately, the original meanings of the terms have become blurred and a variety of definitions and underlying philosophies are attached to the trophic state terms. Some of these definitions bear little resemblance to the original concept.
Indices of Trophic State
One lingering artifact of the multiple variable trophic state definition of Naumann-Thienemann is the idea that trophic state, however defined, cannot be measured directly. Instead, one must resort to indices that reflect or correlate with the “true” trophic state of a waterbody. Numerous indices have been proposed, either in attempts to define trophic state or to act as indicators.
Single Variable Indices
Some contemporary classification schemes use only a single variable to define the trophic state of lakes. This use of a single variable simplifies the classification procedure considerably because only one variable has to be measured. But which variable is the proper one on which to base the classification? Phosphorus loading, phosphorus concentration, chlorophyll concentration, algal productivity, algal biomass (often estimated via Secchi depth and/or chlorophyll concentration), and hypolimnetic oxygen deficits have all been proposed at one time or another for a single variable index. Each could be a valid candidate for trophic state classification and are briefly discussed below.
Phosphorus Loading
Vollenweider (1968a) radically changed our view of lakes when he emphasized the importance of nutrient inputs from the watershed in the determination of the concentration of nutrients and, ultimately, the density of algae in the lake. As we have seen, the idea of the impact of watershed characteristics originated with Naumann, but, only 50 years later was Vollenweider able to convince a new generation of limnologists to look to the watershed to understand the lake.
Hutchinson (1969) and Odum (1969), emphasized the importance of the watershed by defining trophic state by the loading of nutrients to the lake. For Hutchinson, trophic state was a description of the potential for a lake to respond to nutrient loading rather than a description of that response. A eutrophic system would be a system in which the total potential concentration of nutrients was high, whether or not it was expressed in a correspondingly high algal or macrophyte density. Odum (1969) and Beeton and Edmonson (1972) defined oligotrophy and eutrophy based on the amount of nutrient loading.
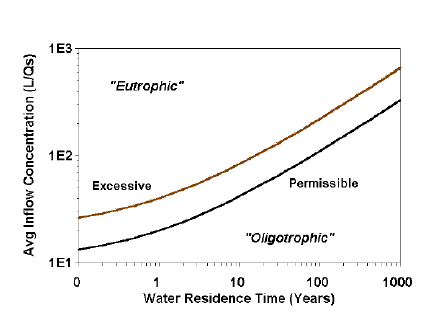
Figure 7.2. A Vollenweider loading graphic illustrating the divisions between “Permissible” (oligotrophic-mesotrophic boundary) and “Dangerous” or “Excessive” mesotrophic-eutrophic boundary) phosphorus concentrations.
A series of loading graphs of Vollenweider (1968, 1975, 1976), appeared to support the idea that trophic state should be directly related to loading. Figure 7.2 illustrates how investigators with loading data are tempted to simply classify the trophic state of a lake. In this case, trophic state is determined by plotting “Average inflow concentration,” which is calculated by dividing loading (L) by water loading (qs), against hydrologic constraints (water residence time). The “Permissible” line is the boundary between oligotrophy and mesotrophy, and “Excessive” line, the boundary between mesotrophy and eutrophy (Vollenweider 1976).
In reality these lines do not represent loading classifications, but instead predict in-lake phosphorus concentrations of 10 and 20 µg/L (Vollenweider 1968, 1971, 1976). Thus, trophic state categories are actually based on the predicted phosphorus concentration in the lake, not loading. The graphs only illustrate the relationship between loading and predicted lake concentration. In practicality, if the investigator wanted to know the present trophic state, he/she could have simply measured phosphorus concentration, and not gone to the trouble of estimating loading. The value of the graphs and the associated models is in the prediction of trophic state if nutrient loading is changed, not the estimation of present trophic state.
Apart from the misuse of the Vollenweider graphs by some to estimate the trophic state of a lake, nutrient loading has not been used extensively to define trophic state. Although the impact of nutrient loading on lake conditions cannot be understated, the use of nutrient loading to define the “state” of the lake seems inappropriate. Loading can only, as Hutchinson implies, define the potential state of the lake. While potential nutrient concentration is an important measure, it does not provide the information about the lake that has attracted limnologists to trophic state classification.
Phosphorus Concentration
The concentrations of nutrients, especially phosphorus, have probably been the most popular variable for single variable trophic state indices. The dominance of trophic state designations based on phosphorus concentration rather than nitrogen is probably the result of the widespread belief that phosphorus limits algal growth in most lakes. The impact of Vollenweider’s loading models on the field of predictive limnology and management also helped emphasize the role of phosphorus.
In phosphorus limited lakes, there should be a strong relationship between phosphorus (usually measured as total phosphorus) and plant (algal) biomass. Because of this relationship, phosphorus can be used as an estimator or predictor of production. If phosphorus concentration is used to predict trophic state (biological production), nutrient concentrations are being used in the manner that Naumann originally intended.
That idea that phosphorus can predict trophic state (production) must be clearly differentiated from the use of phosphorus as the definition of trophic state. While Naumann considered phosphorus and nitrogen to be the primary factors that determined trophic state, he initially based trophic classification on the biological condition of the lake, not the cause of that condition. The trophic state of a lake is a biological condition caused by various factors such as nitrogen and phosphorus, but also potentially affected by pH, turbidity, color, etc.
If phosphorus is used instead of production to define the trophic state of a lake, the program coordinator must be willing to forgo any accurate inference of the biological condition in a large number of lakes and reservoirs. Even the best of the chlorophyll-phosphorus models has considerable variation. More importantly, in some lakes, and certainly in many reservoirs, algal chlorophyll is not related to phosphorus concentration. In turbid lakes and reservoirs, for example, chlorophyll cannot be predicted with any accuracy because much of the phosphorus is probably attached to the non-algal particles and is unavailable for algal growth (Carlson 1992). If the biological condition of the lake is of any importance to the classification or management of a lake or reservoir, there is no justification, neither historical nor practical, to use phosphorus as the defining variable of trophic state.
Algal Productivity
Primary productivity, the rate at which light energy is incorporated into plant cells, has long been a standard of trophic state classification. Åberg and Rhode (1942) and Rhode (1969) interpreted Naumann’s original trophic state definitions to be the biological productivity of a lake rather than production (the amount of plant material in the lake). Certainly, the biological dynamics of a lake and, to some extent, its biological structure, are dependent on productivity.
Although productivity is an important measure in lake evaluation, and has some historical claim as an early trophic state definition, it does have methodological drawbacks that argue against its use as the major estimator of trophic state. Traditionally, productivity requires an estimate of annual productivity, the obtaining of which requires extensive year-long sampling.
The sensitivity of available methods for data collection is also a problem. The oxygen evolution technique is relatively easy to use and requires little equipment, but is not as sensitive as the Carbon-14 technique, which requires radioactive materials and skilled personnel. There is also the concern about radioactivity in the environment, which makes the ability to use C-14 in the field difficult if not impossible. Productivity is also subject to interpretational problems, because, when it is expressed on an areal basis (mg/m2/yr), productivity can be as much influenced by non-algal attenuation of light as by the rate of plant photosynthesis. When productivity is expressed on a volumetric basis (mg/m3/yr), the effective volume (epilimnetic, photic, or whole lake volume) becomes problematic (Carlson 1979).
Algal Biomass
Algal biomass is the weight of the algae in the lake and should be expressed as concentration. It can be reported as grams of dry weight, grams of carbon, or as biovolume (the total volume of plant material per volume of water). Unfortunately there is no test that can, without error, measure what we glibly call biomass. Variables such as total particulate carbon or total suspended solids can neither differentiate living from non-living materials such as sediments, clays, or detritus; nor bacteria from the algae. Consequently, each of these variables becomes an estimator of biomass, but none can be used to define it. Biovolume does not suffer from problems of interference, but it does involve a time-consuming measurement process which requires some expertise. It must also be converted into carbon or mass units, using some conversion factor.
Two other variables commonly used as algal biomass surrogates are chlorophyll concentration and Secchi depth. The concentration of the plant pigment, chlorophyll, is often used as an indicator of trophic state. Chlorophyll is popular because it is relatively easy to measure and does not suffer from the interferences (detritus and non-algal particulates) found in the other variables. Since chlorophyll is also integral to photosynthesis, chlorophyll serves as the link between productivity (rate of carbon incorporation) and production (biomass).
Chlorophyll has drawbacks as a biomass surrogate, however. Perhaps the greatest drawback is that the amount of chlorophyll in an algal cell may vary considerably, depending on the physiological condition of the cell or the plant species. Cells that are subject to low light conditions will have more chlorophyll in them than cells exposed to high light. Different species of algae will contain differing amounts of chlorophyll (Tolstoy 1979). Despite variation, relationships between chlorophyll and algal biovolume and algal density exist, suggesting that chlorophyll changes as cell density changes (Carlson 1980; Watson, et al. 1992).
Secchi depth has also been widely used as a surrogate estimator of trophic state. Transparency itself is not considered to be a definer of trophic state, but transparency is influenced by algal density, and therefore can be used as an inexpensive surrogate for algal biomass. Secchi depth correlates best with algal biomass (as measured by chlorophyll) in non-colored, non-turbid situations where algae dominate the attenuation on light. However, it is subject to a number of interferences and methodological problems (See Secchi Depth chapter). Thus Secchi depth should not be used exclusively when better methods are also available.
A major oversight in the use of algal biomass as the definer of trophic state is that it ignores the presence and importance of aquatic macrophytes in the determination of the trophic state. Their omission may seriously underestimate the total plant biomass in a lake. A method to remedy this problem will be discussed later.
Hypolimnetic Oxygen
The presence or absence of oxygen in the hypolimnion of lakes is often used as a major aspect of trophic state classification. When Naumann’s and Thienemann’s classification schemes were combined, hypolimnetic oxygen became one of the defining characteristics of trophic state. As mentioned earlier, problems arose when morphometric factors were found to also play a major role in defining the presence or absence of hypolimnetic oxygen during the summer. Hypolimnetic temperature was also found to regulate the rate of oxygen depletion. Dissolved organic compounds also contributed to the depletion of oxygen, even in lakes of low productivity, and thus became one of the defining characteristics of dystrophy (Thienemann 1921; Naumann 1932).
Some limnologists would use the presence or absence of oxygen as the sole delimiter of the difference between oligotrophic and eutrophic lakes, regardless of the productivity or production within the lake. While it is true that oxygen depletion can have a dramatic effect on the biota and chemistry of the lake, it would be better to consider hypolimnetic anoxia as a possible result of the lake’s trophic state, not the definition of it. Certainly, anoxia can result from increased productivity, but the relationship between the amount of productivity and anoxia is modified by the volume of the hypolimnion and the temperature of the hypolimnetic water. Volume and temperature have nothing to do with the trophic state concept, yet are major determinants of hypolimnetic anoxia. Thus the term, trophic state, loses much of its meaning if temperature and volume become its determinants. It seems best, therefore, to regard anoxia as a consequence of eutrophication, relying on Naumann’s, rather than Thienemann’s trophic concept.
Multi-Variable Definitions
In the combined Naumann-Thienemann trophic state definition, the lines between morphological factors, chemical factors, and biological structure became increasingly blurred. Naumann’s original emphasis on biological production became lost in a list of “trophic state” variables, some or all of which were used to classify lakes. Some of the modern treatments of trophic state continue this “combination-of-ingredients” approach to trophic classification, declaring that trophic state is a complex aggregate of physical, chemical, and biological variables that can only be dealt with in a multivariate manner and classified in probabilistic terms. Some of the most prevalent forms of multi-variable classifications are discussed below.
Cause-Effect Combination Definitions
The simplest forms of these multifaceted definitions are those that combine a nutrient causal factor, usually phosphorus, with a biological effect factor, such as algal biomass. For example, a eutrophic lake would be a lake with high algal chlorophyll caused by high phosphorus concentrations or high nutrient loading. In these definitions the process of eutrophication, rather than trophic state, is being defined. For example, eutrophication might be defined as increased nutrient loading (cause) which results in increased biological productivity, plant biomass, or hypolimnetic anoxia (effect). Carlson (1984) points out that these cause-effect definitions suffer because, in some cases, the effect is not necessarily the result of the defined cause. Zooplankton grazing or short water residence times can lower algal density despite high nutrient loads. If a lake becomes shallower with time, and macrophytes become abundant, has the lake undergone eutrophication if plant productivity has increased but external loading or internal nutrient concentrations have not?
This linking of cause and effect triggered the proliferation of Naumann’s terminology, and the weaknesses and pitfalls of that approach remain. Faced with situations where the effect occurred independent of changes in the causal factor, the investigator must either ignore the contradiction, choose to classify on either the cause or the consequence, or, as did Naumann, add new names to the system. None of these options seems desirable.
Classifications Based on Lake Types
One unfortunate remnant of the Naumann-Thienemann trophic state system is the belief that lakes can be divided into distinct classification groups or “lake types.” Lake typology reflects the philosophy that lake types are distinct, potentially separate entities, each able to be classified according to its own characteristics (Carlson 1979). These characteristics may exhibit variation within each lake type, and may even overlap between types, but the types are considered largely distinct from one another.
Often typological classifications use a table of characteristics that would be expected for each lake type. An example of a qualitative list of characteristics is given in Table 7.2. This list provides a general impression of the trophic state of a lake or reservoir, but is difficult to use. Quantitative tables also exist, using either distinct boundaries between trophic states or overlapping ranges of values, as in Table 7.3.
Usually the variables on a quantitative or qualitative list are not given any priority; a lake may be classified as oligotrophic by one variable and mesotrophic or eutrophic by another. This situation often happens in deep lakes where the hypolimnion contains oxygen but there are visible algal growths in the upper waters, or in smaller lakes, where the water may be clear, but the hypolimnion is anoxic.
These indices are assumed to work no matter what the kind of lake or where it might be located. Even Naumann and Thienemann recognized that lakes vary geographically in their characteristics. As mentioned earlier, Thienemann initially thought that the classification system was only suitable for lakes in temperate climates.
These classification systems produce only a qualitative label for the reservoir, however. Labels do not lend themselves to quantification, and, therefore, to prediction. Thus, the trophic designation becomes a dead-end, rather than a gateway into predicting the other aspects of the reservoir’s chemical and biological condition, as was envisioned by Naumann.
Qualitative labels also suffer because the labels do not recognize variations within the type. There is a great deal of variation and change possible in any variable, be it phosphorus, chlorophyll, or biological structure, that is incorporated within the single trophic state designation of “eutrophic.” Under the present trophic state classification system two lakes of the same trophic label might have chlorophyll concentrations that vary by two or four-fold. Most limnologists don’t recognize this as a problem, so how could volunteers be expected to know that these lakes might function very differently?
One solution is to add more trophic categories, such as ultra-oligotrophic and hypereutrophic, but this adds more names to memorize and to characterize. Adding more categories also undermines the concept that lakes are distinct types, and tacitly acknowledges that the names are being used to arbitrarily divide a continuum.
List-of-characteristics approaches also ignore all of the other trophic types that Naumann erected. Lists such as that given in Table 7.2 could not classify a lake with a 10 cm transparency filled with silt nor a lake with highly colored water. Of course the list could be expanded to include other lake types, but the list approach was tried by Naumann and history discarded it. It does not seem necessary to recycle an approach proven to be inadequate.
Probabilistic Indices
A quantitative typological classification system was developed by a study group sponsored by the Organization for Economic Cooperation and Development (OECD). The study group apparently believed that lake types did exist, but that there was variation in the values of variables associated with any lake type. They produced a classification system based on the probability that a lake or reservoir will have a given trophic state (Fig. 7.3). For example, a lake having a total phosphorus concentration of 10 mg/m3 in the epilimnion would have a 63% percent probability of being oligotrophic, a 26 percent chance of being mesotrophic, and a 1 percent chance of being eutrophic (Vollenweider and Kerekes 1980). Contrast this approach with that of Vollenweider (1976), where 10 mg/m3 is used as an absolute boundary between oligotrophy and mesotrophy.
The assumed advantage of the probabilistic approach is that responses to a given variable, such as phosphorus, will vary lake-to-lake, and therefore prediction of its trophic state is best stated in probabilistic terms. This approach is analogous to the difference of predicting a single phosphorus concentration for a lake from a loading model and the presenting the probability of a predicted nutrient concentration.
However, there are several problems with the OECD classification method. First, the system is based on the premise that distinct lake types exist. The probabilistic advantage exists only if distinct trophic types actually exist. However, the criterion or criteria that designate a given trophic type are not stated. What does an oligotrophic lake look like at high phosphorus concentrations? Is it called oligotrophic because of low biomass, productivity, or, because oxygen remains in the hypolimnion?
The original designations were based on the opinions of experts, not on a single or even multiple quantitative criteria. The ranges of the curves were obtained by deriving a normal curve from the mean and standard deviations of opinions of a group of experts; no lake may ever exist at the extremes of each trophic state. Setting probabilistic limits may seem more realistic than absolute limits, but only if trophic states are real, not arbitrary divisions of a continuum.
A second problem with this approach is that, as with a qualitative classification system, no effort has been made to first correlate the different variables to provide a single trophic designation. It is possible that a lake may be classified as eutrophic if phosphorus is used as the defining variable, and mesotrophic or even oligotrophic if chlorophyll is used. This dual classification is not due to variability in the values of each variable, but to the fact that the authors of the index did not examine possible empirical relationships between variables.
Finally, the end product of the index is a trophic state label. Apparently all the sources of variation are incorporated in the probability distribution; no effort is made to identify and separate out the sources. Therefore, this type of index has little predictive ability, especially outside the geographical region where it was designed. The index suffers from all the problems listed above for qualitative indices.
Quantitative Multivariable Indices
Some indices attempt to maintain the multiple variable aspect of the Naumann-Thienemann concept while adding a quantitative trophic state designation to the index. This is done by combining more than one variable into a single index value. The multivariate indices of Brezonik and Shannon (1971) and the EPA trophic state index (EPA 1974) utilize a number of variables related to trophic state, yet deviate from the classical interpretation in that they produce a continuous, numerical classification rather than typological categorizations.
The drawback of indices such as these is that several variables must be measured before a classification can be made, increasing the expense of classification. These indices also have limited utility in providing classifications if data are missing. They cannot be used for predicting future states because all of the variables cannot be predicted independently. Refer to Carlson (1979) for a detailed description and discussion of these indices.
Trophic State: An Evaluation
Numerous definitions of trophic state now exist in the literature. There are so many, in fact, that the entire concept is at risk of becoming a “non-concept.” Carlson (1984) comments that this situation would be a real loss to limnology, because the concept, if not the classification, still has the potential to communicate valuable information to scientists and to the lay public. Perhaps even more importantly, it has the ability to aid in the organization of thoughts, ideas, and research about lakes. There are good reasons to think that trophic state remains a critical organizing concept in the study of lakes.
Imagine asking questions about fish production without referring to the base of food chain, or discussing restoration without reference to the watershed. Most investigators will preface their studies with a description of the trophic state of a lake, assuming that all the readers will understand the meaning of the term. Trophic classification is a necessary statement that allows us to locate the lake in the production continuum. From that location we can make predictions of structure and function of that system. Without that location and the ensuing ability to estimate further attributes of the system, each lake becomes an independent entity, devoid of all connection to previous information and knowledge of other lakes, or even previous studies. This certainly is not the way most limnologists think of lakes and limnology.
Any attempt to resurrect and modernize the Naumann-Thienemann trophic concept will ultimately be met with ever-increasing frustration similar to that found by past investigators. Some classifiers have chosen to ignore the possible variations in biological structure caused by factors other than nutrients, producing a single-axis trophic scale based on nutrients alone. Unfortunately, the biological structure of all lakes does not respond in a linear manner to nutrient additions. If the investigator chooses to include other abiotic axes as well, he risks the proliferation of lake types, overlapping and contradictory classifications, and a system as cumbersome and as susceptible to collapse as was the Naumann-Thienemann model.
At the risk of producing just such a trophic classification system, below are several statements which may be controversial, but might lead to a usable and useful classification system.
1. There are no such thing as lake types.
Lake typology is a historical artifact that has no reality in lakes. No study supports the concept that lakes or their biota have a distinct identity or wholeness that separates one lake type from another. Most abiotic variables change continuously along gradients, and production and the biological structure change continuously as well. The propensity to lump lakes into groups is a quirk of the human mind, not a necessity determined by Nature.
The presence or absence of hypolimnetic oxygen comes closest to a single separating variable because along the entire axis of possible nutrient and algal concentrations, anoxia develops relatively rapidly (within one doubling of phosphorus concentration). However, even the development of hypolimnetic anoxia is a continuous, rather than a discrete process, appearing to be abrupt only when compared to the entire spectrum of possible nutrient or biomass concentrations. In any given lake, the process is defined as the rate at which oxygen is depleted, not the mere presence or absence of oxygen.
Dividing a continuum into subgroups is done for convenience. However, once classes have been established, they take on a life and reality of their own, as happened with Naumann’s classification. It would be best to abandon the trophic terminology all together, relying on quantitative classifications instead. It is more difficult to ascribe groupings to a numerical scale.
2. Lake typology and trophic classification are not the same thing.
As the original trophic classification developed, the original classification of production became less and less obvious as the principal variable of interest. For the most part, the classification exercise became one of classifying lakes rather than classifying the amount of biological production. All the factors that affect production became incorporated into the classification; nutrients, light, morphology, turbidity, location, etc.
Lakes can be described in many ways, including depth, thermal structure, water chemistry, size, etc. Trophic state, however defined, is only one possible manner of classifying lakes. In retrospect, Naumann’s terminology should have been specific to production or to the factors that limit production, not incorporate both. An acid lake is only acidotrophic if acid limits production. A turbid lake is argillotrophic only if the turbidity limits plant biomass. Unfortunately, Naumann’s classification seemed to confuse, then fuse, trophic description with the description of the lake. If trophic classification is looked upon as reflecting one particular (and important) aspect of a lake rather than as some sort of total classification, the trophic concept can be kept simple and in perspective.
3. Production is the simplest and most useful definition of trophic state.
Much of what is written in this chapter alludes to the fact that no single variable or even concept has exclusive historical claim to be the “true” definition of trophic state. Even in Naumann’s time, and apparently with his blessing, the concept changed and became more elaborate. The fact that the classification finally collapsed indicates that the scheme had become too elaborate and inclusive. It no longer reflected a chief purpose of classification—utility. Many biological classifications, including binomial nomenclature, are, at least in part, artificial. Being largely a construct of the human mind, they are simple tools in organizing and communicating ideas. Trophic state definitions are no different; they must organize knowledge and they must communicate that knowledge.
It has already been explained why nutrients should not be used as the basis for a trophic state definition. It would not be historically accurate, but more importantly, neither phosphorus nor nitrogen is the primary object of interest in lake classification or management. They only gain their importance as they relate to or affect the biological situation in the lake. The fact that a lake has high concentrations of phosphorus is only of interest if that phosphorus can determine the amounts of algae or macrophytes.
Definitions based on either biomass or productivity can draw upon a long tradition of classifying aquatic as well as terrestrial ecosystems by amount of organic matter in the system and/or the rate of entry of energy into the system. The reasons why productivity would not be the best trophic state variable have already been explained; it is difficult to measure and to interpret.
Biomass-related trophic state definitions are consistent with systems terminology’s use of the term “state,” as a measure of the amount in a system at a given point in time. Although the term “biomass” itself must be operationally defined, it can be estimated by a number of techniques, from Secchi depth to adenosine triphosphate (ATP) analysis. With such a variety of available techniques, a technique appropriate to each budget or situation can usually be found. Biomass also lends itself to multi-lake surveys, where there is often insufficient time to do intensive productivity analyses. Finally, biomass is an approximate measure of the problems that plague lakes. Few citizens complain about the productivity of their lake and fewer yet lodge complaints about phosphorus. A biomass-related trophic state definition places the emphasis of the classification on the problem (e.g., too much algae or too many macrophytes which, in turn, interfere with lake uses) rather than on any potential cause.
4. Eutrophication is nothing more (or less) than the movement of a lake’s production along a continuum in a direction from oligotrophy towards eutrophy.
In Naumann’s trophic state classification, the terms oligotrophic and eutrophic marked two classes of lakes along the nitrogen and phosphorus axis. As a lake became eutrophic, the process was called eutrophication. Conversely, if a eutrophic lake became oligotrophic, the process could be called oligotrophication. Eutrophication is not a mysterious process or even one necessarily linked to nutrient change. It is simply a directional movement.
Because production might be increased or decreased by factors other than nutrients, it is more appropriate to use production as the central axis rather than nutrients. Because production is most often related to changes in nutrient status in the lake, eutrophication and oligotrophication are processes often caused by changes in the lake’s nutrient content, and, ultimately, nutrient loading to the lake. However, the change could just as well take place if another limiting factor, such as turbidity, were removed, as long as sufficient nutrients were available to allow increased plant production. Eutrophication would have occurred even if there had been no change in loading, or even nutrient status.
5. Biological structure of lakes is affected by many factors other than nutrients and should not be used to define trophic state.
Unlike production, the biological structure of a lake is much more susceptible to change along gradients other than nutrients. As mentioned above, one mechanism to maximizing production and productivity is a change in the dominant species to those best suited to the ambient conditions. Changes in species can be expected as a lake becomes acidic or colored with humic substances. In other cases, intolerance to prevailing conditions will eliminate whole groups of organisms, as was found in acidic lakes, where vertebrates were largely missing. In these instances, whole trophic pathways will be altered or eliminated. The same alteration of food webs may also be found in other environments such as turbid reservoirs and lakes.
If trophic state were defined on the basis of biological structure rather than on production, it might be expected to change as a function of the intensity of a number of environmental factors. Change pH, nutrient loading, hypolimnetic anoxia, or salinity, and the biological structure (and therefore trophic state) will be expected to change as well.
Clearly, structural changes will not fit neatly into lake types because changes will probably occur gradually along any one or more environmental axis. Any sort of typological classification would be impossible; the name game would be overwhelming. Probably some sort of multivariate quantitative classification would be most useful. Whatever the approach that is used, the ability to classify the biological structure of lakes is clearly needed.
Biological structure has a legitimate and compelling claim to the concept of trophic state. Although not the original trophic state definition, biological structure gained importance in later classifications, especially after the merger of the Naumann and Thienemann classifications. Naumann considered that many of the lake types, such as acidotrophy or argillotrophy, had equal productivity but distinctly different biological communities.
In a subtle way, Naumann made a distinction between production and structure. He split the classification into two typologies, one describing the water (Wasser) and the other the water body (Gewasser). In the first he placed production, in the second, biological structure. What he did was to distinguish between variables such as production and productivity and those relating to biological species (structure). This distinction is useful because they are really two distinct but related aspects of biological systems.
Production and productivity relate to the amount and rate at which energy enters the lake system. They share with other variables, such as nutrient concentration, the attribute that they are conservative, that one can always account for the income, outgo, and location of every kilocalorie or gram. People can write energy or nutrient budgets for them and predict how much energy or nutrient will be used in the future. They are, however, fundamentally different from biological structure. Biological structure is based on biological species. A species becomes present in the community when a single organism arrives, but the loss of a single individual does not mean the species is absent. For a species to be absent again, it must go extinct. A species cannot be weighed; it may consist of a single individual weighing a kilogram, or the total weight of millions of individuals of a species may weigh only a thousandth of a gram.
Species are a member of a category called information. Information is non-conservative; that is, you can’t weigh or account for information in the manner you can with materials and energy. Two cents worth of information can be given to one person or a million people, each receives the same amount of information. A single individual of a species brings far more than two cents worth of information into an ecosystem. That information is present whether there be one or a million individuals of that species. This non-conservative nature of information makes it difficult, if not impossible, to predict species structure from energy or material variables alone. A kilogram of organic material may contain a single or a thousand different species, arranged in an almost infinite number of trophic web arrangements.
More important yet, biological structure has the unique ability to adapt, evolve, and change to fit the surrounding environment. Given time, it is theoretically possible for life to evolve for the maximum utilization of nutrients and energy even in the most extreme environment. Within broad thermodynamic constraints, however, evolution can produce a dazzling kaleidoscope of possible biological communities.
If biological structure can be so diverse, it could be successfully argued that each lake ecosystem is to some extent composed of a unique community structure. If each lake is different from the next, then any classification ultimately will be frustrated by this range of possibilities. Alternatively, classification may attempt to force the diversity of structure into an artificial classification scheme, overlooking the small differences, preferring to see the similarities.
Biological structure classification is distinctly different from what Naumann originally intended (i.e., the linking of watershed characteristics to the biological production of the water). The original trophic scheme was deeply involved in the factors of production, not structure. It is to Naumann’s credit that he insisted that production would, in turn, affect structure, and ultimately the ontogeny of the lake itself. Classification based on biological structure would have to include numerous measures that affect biological structure, but which are unimportant to Naumann’s concept of factors that affect production.
In the final analysis, it is recommended that the amount of plant production (biomass) and not biological structure be used as the definition of trophic state. It has historical consistency, is relatively easy to measure, and is a measurement that conveys meaning to limnologists, lake managers, and the public. Biological structure should be considered as a separate classification. Structure is important and far more vulnerable to environmental stresses than production. The ability to classify and track structure is an important aspect of limnology because structural changes may ultimately affect function. It deserves more attention, but it should not be called trophic state. Call it biodiversity, biological structure, biotype, or whatever, but just not trophic state.
Trophic State and Volunteer Programs
The coordinator of a volunteer program is in a unique position to understand the need for a clearly defined, useful, and communicable definition for trophic state. The coordinator serves at least three basic constituencies. First, monitoring programs are often mandated by the state governments. In some states coordinators are required to obtain certain information to satisfy 305(b) reporting requirements and to qualify for 314 Clean Lakes funds. The trophic state determination is needed for both programs. The more complex the definition of trophic state, the more information that will be required to classify lakes. If the definition is too complex, data requirements will be beyond the scope and budget of most volunteer monitoring programs.
Second, volunteers expect information, not labels. They will be poorly served for their time and effort if all they receive is a comment that their lake is eutrophic. What does this term mean to the volunteer? Is the lake in good or bad condition? Should it be restored or protected? What is the prognosis for recreation and other uses? It would be better if the definition reflected a single aspect of the lake very well rather than present a combined variety of factors that does not convey quality information.
Third, the coordinator, or those that use the volunteer data, must make management decisions. Decisions require information. However, if the goal of the program is serving the data collection needs of a classification system, that classification had better provide more information than just a name. Certainly trophic state is only one facet of what should be called water quality, but it would be ideal if the definition described an important aspect of a management program.
The trophic state model presented above is amenable to a tiered level-of-effort approach which is compatible with the funding and goals of many volunteer programs. Most programs begin with a Secchi depth monitoring program because it is easy to train volunteers in the disk’s operation and because Secchi disks are relatively inexpensive. Since Secchi depth is also an indicator of algal biomass, it is also possible to make estimates of trophic state, albeit with considerable uncertainty.
As a program matures, chlorophyll pigments and total phosphorus are added to provide a better estimate of biomass. Later in this chapter, a method will be given that uses Secchi depth, chlorophyll, and total phosphorus to make inferences about potential limitation. Addition of total nitrogen and estimates of macrophyte biomass would complete this suite of variables that would completely estimate trophic state, as defined by plant biomass, and the nutrient factors that often cause that trophic state.
Additional sampling by volunteers could provide information on biological structure. Samples of macrophytes, phytoplankton, and zooplankton could be obtained by the volunteer and shipped to a laboratory for identification. Creel censuses or careful records of fishing success by the volunteers might give some indication of fish community structure.
From these data, a picture of a lake’s trophic and biological structure will emerge. From that picture, it may be possible to ask questions of causation, and a watershed monitoring program could be initiated. Volunteers could do much of the work in a watershed monitoring program and the information gathered could be very useful in managing the lake.
Finally, the information can be combined into a regional picture of trophic state. This regional aspect of trophic state was initially envisioned by Naumann and today can serve as a goal of statewide monitoring. Its use as a method for answering and generating questions has yet to be fully explored.
Recommendations
Trophic state determination is an important aspect of lake surveys. Trophic state is not the same thing as water quality, but trophic state certainly is one aspect of water quality. Of particular interest for volunteer programs is that trophic state determination can be performed by volunteers and it can be immediately useful to them. Several recommendations can be made with regard to the use of trophic state classifications in volunteer programs.
- Use the simplest definition of trophic state: neither you nor the volunteer will benefit by making the concept complex or somehow mysterious.
- The recommended definition is plant biomass: it is historically correct, simple to measure, and simple for a volunteer (or manager) to understand. It also can be predicted from nutrient models and can be used to predict other biological characteristics.
- The trophic state index of Carlson (1977), which is based on plant biomass, is recommended as the simplest method of relating trophic state concepts to volunteers.
- Remove the mystery from the term eutrophication. Rather than linking the process to nutrients, which can cause all sorts of interpretational problems, simply define it as a movement of the lake’s trophic state in the direction of more plant biomass. The definition is simple and far more functional than any other definition.
- Use trophic state as a teaching tool. Discuss with the volunteers all the possible factors, not just nutrients, that could make a lake more eutrophic. For example, explain that the deposition of erosional materials will cause the lake to become shallower, and therefore enhance macrophyte growth, thus affecting the total amount of biomass. Discuss the ramifications of change in plant biomass, how it affects hypolimnetic oxygen and fish species and its possible effect on food chains and recreational potential.
- Be very careful about using quality terms when speaking of trophic state. Even your own perception of quality is affected by your background and education. Be sensitive to the fact that not all users will want the same type of water quality that you do. Not everyone considers the ideal lake to be clear. Always be sensitive to the background and needs of the users.
Literature Cited
Åberg, B. and W. Rhode (1942). Uber die milieufaktoren in einigen sudschwedischen seen. Symb. Bot. Ups. 5(3):1-256.
Barica, J. 1980. Why hypertrophic ecosystems? Opening remarks. pp. ix-xi. In: J. Barica and L.R. Mur (Eds.) Hypertrophic ecosystems. Dr. W. Junk bv. The Netherlands.
Beeton, A.M. and W.T. Edmonson. 1972. The eutrophication problem. J. Fish. Res. Bd. Can. 29: 673-682.
Brezonik, P.L. and E.E. Shannon. 1971. Trophic state of lakes in north central Florida. Research Project Technical Completion Report. OWRR Project No. B-004-FLA., Publ. No. 13., Water Resources Res. Center, Univ. Florida.
Canfield, D.E. Jr., K.A. Langeland, M.J. Maceina, W.T. Haller, J.V. Shireman, and J.R.Jones. 1983. Trophic state classification of lakes with aquatic macrophytes. Can. J. Fish. Aquat. Sci. 40: 1713-1718.
Carlson, R.E. 1977. A trophic state index for lakes. Limnology and Oceanography. 22:361-369.
Carlson, R.E. 1979. A review of the philosophy and construction of trophic state indices. p. 1-52. [In] T.E. Maloney (ed). Lake and Reservoir Classification Systems. U.S. Environmental Protection Agency. EPA 600/3-79-074.
Carlson, R.E. 1980. More complications in the chlorophyll-Secchi disk relationship. Limnology and Oceanography. 25:378-382.
Carlson, R.E. 1981. Using trophic state indices to examine the dynamics of eutrophication. p. 218-221. In: Proceedings of the International Symposium on Inland Waters and Lake Restoration. U.S. Environmental Protection Agency. EPA 440/5-81-010.
Carlson, R.E. 1983. Discussion on “Using differences among Carlson’s trophic state index values in regional water quality assessment”, by Richard A. Osgood. Water Resources Bulletin. 19:307-309.
Carlson, R.E. 1984. The trophic state concept: a lake management perspective. p. 427-430. In: Lake and Reservoir Management: Proceedings of the Third Annual Conference of the North American Lake Management Society. U.S. Environmental Protection Agency. EPA 440/5/84-001.
Carlson, R.E. 1992. Expanding the trophic state concept to identify non-nutrient limited lakes and reservoirs. pp. 59-71 [In] Proceedings of a National Conference on Enhancing the States’ Lake Management Programs. Monitoring and Lake Impact Assessment. Chicago.
Cooke, G.D. and R.E. Carlson. Water quality management in a drinking water reservoir. Lake and Reservoir Management. 2: 363-371.
Hutchinson, G.E. 1969. Eutrophication, past and present. In: Eutrophication: Causes, Consequences, Correctives. National Academy Sciences.
Hutchinson, G.E. 1973. Eutrophication. Am. Sci. 61: 269-279.
Järnefelt, H. 1927. Ein jurzer ueberblick ber die imnologie Finnlands. Verh. Internat. Verein. Limnol. 4: 401-407.
Kratzer, C.R. and P.L. Brezonik. 1981. A Carlson-type trophic state index for nitrogen in Florida lakes. Water. Res. Bull. 17: 713-715.
Lundbeck, J. 1934. ber den primar oligotrophien Seetypus und den Wollingster See als dessen mitteleuropaischen Vertreter. Arch. Hydrobiol. 27: 221-250.
Naumann, E. 1919. Nagra synpunkter angaende limnoplanktons okologi med sarskild hansyn till fytoplankton. Sv. Bot. Tidskr. 13: 129-163.
Naumann, E. 1929. The scope and chief problems of regional limnology. Int. Revue ges. Hydrobiol. 21: 423.
Naumann, E. 1931. Der pH-Standard des Süsswassers. Verh. Internat. Verein. Limnol. 5: 291-304.
Naumann, E. 1932. Grundzuge der regionalen Limnologie. Die Binnengewässer. 11:1-176.
Odum, E.P. 1969. The strategy of ecosystem development. Science. 164: 262-270.
Oglesby, R.T., J.H. Leach, and J. Forney. 1987. Potential Stizostedion yield as a function of chlorophyll concentration with special reference to Lake Erie. Can. J. Fish. Aquat Sci. 44(Suppl. 2): 166-170.
Osgood, R. 1983. Using differences among Carlson’s trophic state index values in regional water quality assessment. Wat. Res. Bull. 18: 67-74.
Rast, W. and G.F. Lee. 1987. Summary analysis of the North American (US portion) OECD eutrophication project: Nutrient loading – lake response relationships and trophic state indices. U.S. Environmental Protection Agency. EPA-600/3-78-008.
Rodhe, W. 1969. Crystallization of eutrophication concepts in Northern Europe. In: Eutrophication: Causes, Consequences, Correctives. National Academy Sciences.
Rodhe, W. 1975. The SIL founders and our fundament. Verh. Internat. Verein. Limnol. 19: 16-25.
Smeltzer, E. and S.A. Heiskary. 1990. Analysis and applications of lake user survey data. Lake and Reservoir Management.
Thienemann, A. 1921. Seetypen. Naturwissenschaften. 18: 1-3.
Thienemann, A. 1926. Tropische Seen und Seetypenlehre. Verh. Internat. Verein. Limnol. 3: 69-71. (This is a summary of a longer paper in Arch. Hydrobiol. Suppl. 9: 205-231).
Tolstoy, A. 1979. Chlorophyll a in relation to phytoplankton volume in some Swedish lakes. Arch. Hydrobiol. 85(2):133-151.
U.S. Environmental Protection Agency. 1974. An approach to a relative trophic index system for classifying lakes and reservoirs. National Eutrophication Survey. Working Paper No. 24.
Vant, W.N. and R.J. Davies-Colley. 1988. Water appearance and recreational use of 10 lakes of the North Island (New Zealand). Verh. Internat. Verein. Limnol. 23: 611-615.
Vollenweider, R.A. 1968. The scientific basis of lake and stream eutrophication, with particular reference to phosphorus and nitrogen as eutrophication factors. Technical Report OAS/DSI/68.27. Organization for Economic Cooperation and Development. Paris.
Vollenweider, R.A. 1975 Input-output models. Schweiz. Z. Hydrologie. 37: 53-84.
Vollenweider, R.A. 1976. Advances in defining critical loading levels for phosphorus in lake eutrophication. Mem. Ist. Ital. Idrobiol. 33: 53-83.
Vollenweider, R.A. 1989. Eutrophication. p. 107-120. In: Meybeck M., D. Chapman, and R. Helmer, [Ed.]. Global Freshwater Quality – A First Assessment. World Health Organization and the United Nations Environmental Programme.
Vollenweider, R.A. and J.J. Kerekes. 1980. Background and summary results of the OECD cooperative program on eutrophication. In: p. 25-36. In: Proceedings of the International Symposium on Inland Waters and Lake Restoration. U.S. Environmental Protection Agency. EPA 440/5-81-010.
Walker, W.W. 1985. Empirical methods for predicting eutrophication in impoundments. Report 3. Phase II. Model refinements. Technical Report E-81-9. US Army Corps of Engineers Waterways Experiment Station, Vicksburg, Miss.
Watson, S., E. McCauley, and J.A. Downing. Sigmoid relationships between phosphorus, algal biomass, and algal community structure. Can. J. fish. Aquat. Sci. 49: 2605-2610.
Weber, C.A. 1907. Aufbau and vegetation der Moore Norddeutschlands. Bot. Jahrb. 40. Beiblatt zo den Botanischen Jahrbuchern. 90: 19-34.